Potential Antiviral Drug for the Treatment of SARSCoV- 2 Based on Quinacrine and Porous Silicon
Introduction
The SARS-CoV-2 pandemic has been going on for several years. The number of deaths in the world from SARS-CoV-2 according to the WHO at the beginning of 2022 is approaching six million people [1]. During this difficult period of time, the development of new antiviral drugs against the virus is necessary. A promising active ingredient against SARS-CoV-2 was synthesized a long time ago as an anti-malaria drug quinacrine (Qx) [2]. It is a well-known small molecule inhibitor of RNA virus replication with known anti-prion activity, also used as a potent Ebola virus inhibitor both in vitro and in vivo. Qx has been shown to be active against SARS-CoV-2 [3]. In the works [4,5], efficacy against SARS-CoV-2 in vitro was demonstrated, with a half-maximal effective concentration (EC50) in the range of 0.58-1.88 mmol. There are several mechanisms of antiviral action [6]. Among them are the following:
1. The ability of quinacrine to intercalate DNA and RNA, thus inhibiting virus replication [7],
2. Increasing the pH of acidic organelles,
3. Qx can inhibit autophagy [3]. Qx is a potent inhibitor of phospholipase A2, reducing cysteinyl leukotrienes and modulating the Th1/Th2 response. Qx may inhibit the secretion of proinflammatory cytokines and Toll-like receptors 7 and 9; molecules involved in the cytokine storm seen in patients with severe disease [3,8]. In [9] it was shown that quinacrine and suramin molecules exhibited a competitive and non-competitive mode of inhibition with IC50 values in the low micromolar range. Surface plasmon resonance (SPR) experiments have shown that quinacrine and suramin alone have moderate to low affinity for SARS-CoV-2 3CLpro, but suramin binding increases the potency of quinacrine by an eight-fold.
At the same time, Qx has a hypotensive effect, cardiac slowing and inhibits myocardial contractility [2,10]. In addition, it is important to gradually release it from the carrier material to smoothly reduce the viral load. The solution to these two problems is possible with the use of porous silicon (por-Si) [11-13], which allows the active substance to be absorbed, delivered to the body and gradually released. For example, since 2010, several patents have been issued in the United States for the use of porous silicon in ophthalmology and immunotherapy [14]. In addition, pure silicon can be loaded not only with medicinal substances, but also with various contrasts, which makes it applicable in theranostics [14- 16]. This work is dedicated to the study of the kinetics of the release of quinacrine from porous silicon for use as an oral drug to reduce the viral load of SARS-CoV-2.
Materials and Methods
To obtain nanoparticles, phosphorus doped monocrystalline silicon <111> wafer with a resistivity 4,5 Ω*cm was used as an initial material. As a method for forming a porous structure on silicon substrates, the electrochemical etching with anodizing current density Ja=80 ma/cm2 and etching duration t=20 minutes was chosen. The choice of such parameters is justified by the fact that the layers obtained under such conditions are the most studied in our laboratory [12,17-20]. After the formation of por-Si layers, to obtain nanoparticles, the plates were placed in a test tube with water, where they were subjected to ultrasonic treatment in an ultrasonic bath (Sapphire, Russia) for 30 minutes. The impact of ultrasound on the etched wafers separates the porous film from substrate and grind the por-Si, as well as to disperse the obtained nanoparticles [12].
The particles were imaged using scanning electron microscopy (Mira Tescan II, Czech Republic). The study of the zeta potential and particle size was carried out on a Zetasizer Ultra laboratory setup (Malvern Instruments Ltd, UK) based on the dynamic light scattering method. Sorption of quinacrine (C
Results and Discussion
It was found that por-Si particles have a negative zeta potential of -5.4 mV. This value of the zeta potential is not too large, and this indicates that the particles can coagulate inbetween. This hypothesis is confirmed by the fact that the results of measurements of the average particle size reached 1200 nm. Figure 1 demonstrates the porous silicon texture typical for the certain type of technological conditions before (Figure 1a) and after (Figure 1b) sonification. As one can see from Figure 1a, there are large pore channels (diameters of macropore channels ≈ 150-180 nm), at the intersections of large pore channels, which divide the main part of the porous layer into blocks of the order of 500-600 nm. We have previously shown by capillary condensation that such porous silicon particles contain micro- and mesopores [21].
This is the most probable points along which fragmentation of the porous layer occurs during ultrasonic treatment. Figure 1b shows por-Si particles after sonification of por-Si layer, as in Figure 1a, with a particle size of the order of 500-600 nm. The method of ultrasonic exposure shows high reproducibility of results, and by adjusting the power of ultrasonic exposure, the size of particles could be varied. The dispersion also contains larger fragments of the porous layer, which is confirmed by the measurements gotten using dynamic light scattering method. Separation of porous silicon particles using size by centrifugation makes it possible to obtain dispersions with a narrower size distribution [12]. As can be seen from Figure 2, porous silicon samples adsorb Qx, which is confirmed by the fluorescence of the corresponding samples.
Figure 1: SEM images of porous silicon before (a) and after (b) Sonification (the inset shows an por-Si particle).
Figure 2: Visualization of porous silicon samples: BKG 1 — background radiation value; ROI 1(BKG 1)—initial suspension of porous silicon powder; ROI 2(BKG 1) — initial suspension of porous silicon powder with adsorbed quinacrine.
The curves for the Qx release from porous silicon particles are shown in Figures 3 & 4 (for Series 1 and Series 2 respectively). As can be seen from both graphs, at the initial time of desorption, the curves do not start from zero, but show some presence of Qx in Krebs-Henseleit solution at t = 0 h. Most likely, this is due to the desorption of that part of quinacrine that was physically adsorbed directly on the outer surface of the porous particles. Then, at further desorption times, both graphs show a linear increasing dependence of optical density on the release time of quinacrine (up to about 3 hours for Series 1 (Figure 3) and up to 4 hours for Series 2 (Figure 4)), then for Series 2, a saturation section is observed. Graphs could be approximated by a function of the next form:
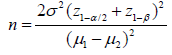
Figure 3: Quinacrine release profile from por-Si particles for Series 1 (experimental values are shown by dots, the solid line approximates the experimental data).
Figure 4: Quinacrine release profile from por-Si particles for Series 2 (experimental values are shown by dots, the solid line approximates the experimental data).
where A0-value of y at t = 0 h, A1-value of y at t →∞, c – a phenomenological coefficient, the value of which will depend on the rate of desorption of a substance from a porous matrix, on the characteristics of diffusion, the composition and temperature of the medium into which the drug is released, and other processes occurring during the release. As one can see from Figure 3, an optical density D=0.0008 at t=0 h is very low, and the amount of sorbed quinacrine is very small. Probably, this is the reason why it is difficult to distinguish the change from linear increasing character to saturation part. The part of linear increasing character of release curves, apparently, is due to the desorption of quinacrine from the internal volume of the porous space of the por-Si particles.
Changing the technological conditions of sorption – increasing the temperature to 600C during the first two hours of sorption led to a significant increase in the amount of quinacrine loading (Figure 4). The optical density increased markedly: by 4 orders of magnitude at initial time of desorption and loading into the internal pores increased by 1-1.5 orders of magnitude. This, in turn, is a good result and makes it possible to use porous silicon as a carrier for the targeted delivery of quinacrine. In this case, a prolonged effect can be achieved: even without the use of special shells, the release of quinacrine is observed for about 4 hours.
Conclusion
The use of porous silicon as a means of delivering quiacrine to the body to reduce the viral load of SARS-CoV-2 has been substantiated. Sorption of quiacrine on porous silicon was carried out and the drug release profiles were obtained. The data obtained allow us to recommend the resulting construct as a candidate for oral use to reduce the viral load of SARS-CoV-2. It has been experimentally shown that sorption of quinacrine by porous silicon particles is observed, as well as its release into the Krebs-Hensleit buffer solution. Increasing the temperature up to 60°C during the sorption process leads to an increase in the efficiency of loading porous silicon particles by ≈4 orders. In addition, prolonged release of it has been achieved.
For more Articles on: https://biomedres01.blogspot.com/
No comments:
Post a Comment
Note: Only a member of this blog may post a comment.