Administration of Ketamine Inhibits Theta and Delta Oscillation During Propofol Induction but Not During Sevoflurane Induction
Background
Ketamine, an N-methyl-D-aspartate (NMDA) receptor inhibitor, given in low doses acts by blocking inputs to inhibitory interneurons, which increases cerebral metabolism [1]. The hypnotic effects are caused by a combination of the immediate blockade of NMDA and hyperpolarization-activated cyclic nucleotide–gated (HCN) 1 channels, and the immediate analgesic effects are probably mediated predominantly by a combination of opioid system sensitization and aminergic antinociception [2]. Ketamine combined with propofol has a shorter recovery time after surgery, with fewer side effects such as nausea, vomiting, and respiratory depression [3,4] Low doses of ketamine may have no effect on bispectral index (BIS) data, [5] but alters the electroencephalogram (EEG) changes of different medications. The disadvantage of using ketamine during surgery is that the depth of anaesthesia cannot be monitored reliably with a BIS monitor because ketamine makes BIS data lack fidelity [6,7]. Different anaesthetic agents cause different pharmacological effects in the human brain, the differences between medications can easily be identified through frontal spectrograms because each medication has individual characteristics as well as frontal–parietal communication disruption [8].
Ketamine increases power density on beta-band neuronal oscillations accompanied by loss of consciousness (LOC) [9]. Slow delta and alpha oscillations are markers of propofol-induced unconsciousness. Sevoflurane has strong alpha and slow delta oscillations at a sub-MAC concentration [1] but sevofluraneinduced unconsciousness was not consistently correlated with anteriorization theory [10]. The appearance of theta oscillations indicates a more profound state of unconsciousness. We can also distinguish the clinical stage of unconsciousness from the power density changes from the high- frequency range to the medical frequency range [11,12]. However, when the patient receives more than one medication, the EEG signals are more complicated than are those of a single medication. The BIS elevation may be due to the arousal mechanism [9] of ketamine in the brainstem and diencephalic arousal centre, or to ketamine selectively binding to NMDA receptors on GABAergic inhibitory interneurons of the pyramidal system [13] causing a disinhibition reaction. The traditional spectral analysis provides simple conclusions that the spectral power of surface frontal EEG increases during anaesthesia initially, followed by a decrease is not detailed enough. EEGs have dynamic features of nonlinearity and non-stationarity.
According to Huang et al. [14], traditional spectral analysis methods based on the assumption that series are linear and stationary fail to meet the characteristics of biological signals. Empirical mode decomposition (EMD) can decompose an EEG into a limited number of intrinsic mode functions(IMF’s) with their own characteristic frequency and amplitude modulations. However, the mode- mixing problem reduces the performance of EMD. To enhance the performance, appropriate masking signals were added into EEG signals to resolve mode mixing [15]. With this method, the frontal EEG showed twice as much energy gathering in IMF2 during general anaesthetic; moreover, anaesthesia agents caused a decrease of power density for low-frequency IMFs [11]. In this study, an enhanced EMD method was used to decompose an EEG signal into a set of IMFs, which preserved the characteristics of corresponding oscillatory components for various frequency bands. The local power for each oscillatory component could be quantified using the root mean square energy of a segment of an IMF. The energy of all IMFs were used to represent multiband effects on EEGs from anaesthesia using ketamine, sevoflurane, and propofol for comparison. We administered analgesic doses of ketamine while the patients were anaesthetized with sevoflurane or propofol. Our goal is to quantitate the EEG changes of oscillations of IMFs, and compare with BIS data during a ketamine propofol anesthesia and ketamine sevoflurane anesthesia.
Methods
Ethics Statement
This study was conducted at National Taiwan University Hospital and was approved by the Institutional Review Board of National Taiwan University Hospital (201508007RINB and 201509018RINB). All participants provided written informed consent after careful discussion of the study methodology.
Clinical Approach: This was a randomized clinical trial. Fifty-five patients aged 20 to 40 years with low anaesthetic risk (i.e. American Society of Anaesthesiologists physical status classification I or II) requiring general anaesthesia were included. The sample size of subgroups was addressed by previous results [7]. In this reference,15 cases in each group with significant results was addressed. We increased the case number in order to decrease the type II error in ANOVA analysis. The participants were assigned by his/her anaesthesiologists to either a sevoflurane group (S) or a propofol group (P) according to surgery requirements. After they were assigned, the method of randomization was by the last number of the patients’ medical chart, if the number is odd, the patient will be randomized to the ketamine group; if the number is even, then the patient as assigned to the control group. Study investigators were not involved in assigning patients to a specific anaesthesia group. In the S group, the patients were assigned randomly to receive ketamine (+K) after LOC or to a normal saline group. In the P group, the patients were assigned randomly to receive ketamine (+K) after LOC or to a normal saline group. For each patient, all standard monitors, including a pulse oximeter, electrocardiograph, BIS monitor, and blood pressure monitor, were set up by independent researcher. All physiological data was connected and recorded to computer prior to anaesthesia induction in the operation room. All patient received 5mcg.kg-1 of alfentanil before induction.
Anaesthetic Protocol: In the S group, we performed inhalation induction by using sevoflurane 3.5 MAC with air at 1.5 L min-1 and oxygen at 1.5 L min-1. When the patient was anaesthetized (indicated by LOC and loss of eyelid response), ketamine 0.5 mg kg-1 (+K group) or normal saline was administered randomly. We ensured that the EEG data were not influenced by noise (e.g. talking), movement (e.g. mask ventilation), or painful stimulation (e.g. intubation) for 3 min after the medication was administered. In the P group, after 1 min of monitoring, an anaesthesiologist began target- controlled infusion induction with propofol targeting a plasma concentration of 4.0 μg mL-1. Once the patients were anaesthetized, ketamine 0.5 mg kg-1 (+K group) or normal saline was administered randomly. We also ensured that the EEG data were not influenced by noise, movement, or painful stimulation for 3 min after the medication was administered. We marked the time of events as the ‘awake baseline’ (T1), ‘LOC’ (T2), and ‘after ketamine injection 3 min’ (T3). The demographic data of each patient and the signal recordings from BIS monitors and the Phillips XP60 were collected. EEG waveforms were collected through a BIS monitor (Aspect Medical System XP platform, a specific electrode strip featuring mainly frontal electrodes).
Data analysis (Flowchart 1)
In this study, EEG signals were recorded at a sampling rate of 128 Hz and decomposed into the first six IMF’s through a masking EMD method. The Data was analysed by lab researcher who is blinded to result.
Enhanced EMD: In the enhanced EMD method, the frequencies and amplitudes of masking signals are determined adaptively according to the natures of signals . A predecomposition using the CEMD method for only IMF1 is performed to determine the frequency and amplitude of masking signals used in the enhanced EMD method. In the enhanced method, a set of IMF’s cI(t) decomposed from a signal x(t) can be derived through the following steps:
1. Derive the predecomposed IMF1 by using the CEMD method.
2. Obtain the time series of the instantaneous frequency and amplitude for the predecomposed IMF1 by using Hilbert transform.
3. Determine the frequency of masking signals by using the mean value of the distribution of the instantaneous frequency, and determine the amplitude of masking signals by using the averaged amplitude of the predecomposed IMF1.
4. Generate four masking signals by using sine waves with the amplitude and frequency determined in step 3. The initial phases of four masking signals are 0, π/2, π, and 3π/2.
5. Add masking signals into the original signal to generate four mixtures for formal decomposition through the CEMD, and decompose IMF1 from each mixture.
6. Obtain a new IMF ci(t) by using the averaged mode function of four IMF1s decomposed from the four mixtures.
7. Obtain the residual by removing the new IMF from the signal for decomposing the next IMF until the residual is monotonic.
Computing Standard Deviation of Original EEG and IMF’s: After computing the Hilbert spectrum of the IMF’s, we compared the instantaneous standard deviation (SD) of the IMF’s and the BIS value. The SD can be used to quantify the root mean square energy of a signal by extended definition. We sought to examine the IMF’s’ energy change according to time; therefore, we used a sliding window technique to derive a time-variant 𝑆𝐷(𝑡) of a signal 𝑆(𝑡), which can be defined as
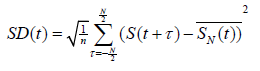
where denotes the mean of 𝑆(𝑡) in the window
The normalized energy of each IMF, which represents the power of each oscillation, was analyzed. Power changes between the awake (T1), LOC (T2), and 3 min post-injection (T3) events in IMF1 to IMF6 were observed and calculated in the patient groups. BIS value of different time point were also collected, and the differences of T1, T2, T3 between groups were analysed. Repeatedmeasurement ANOVA was applied to evaluate between-group effects, and statistical significance was evaluated using SPSS, with p < 0.05 indicating a significant difference between groups.
Results
The result of the demographic data is presented in (Table 1). There are no differences between the four groups in height (HT), blood pressure (SBP, NBP), heart rate (HR), saturation (SpO2), and the mean frequencies of IMF1 to IMF6. The EEG data and demographic data were collected 2 minutes before induction. According to the spectrum of EEG bandwidths and frequencies in clinical use and in research (Figure 1), IMF1 represents γ band neuronal oscillation (>30 Hz); IMF2 represents β band oscillation (13 ± 30 Hz); IMF3 reflects α band oscillation (8– 13 Hz); IMF4 reflects θ band oscillation (3.5–8 Hz); and IMF’s 5 and 6 represent δ band oscillation (0.5 ± 3.5 Hz).
Part 1: We compared the gamma energy and delta energy of the P+K group with that of the P group. The gamma energy significantly increased after ketamine injection (T3) (p = 0.0008). The gamma energy after the patients lost consciousness (T2) was higher by 13%, and increased to 26% at T3 in the P+K group. By contrast, the control group maintained the same level of gamma energy. Both groups exhibited the same increasing trend in beta energy. The elevation of alpha energy at T2 did not differ between groups. The theta energy showed different results (Figure 2). Ketamine decreased in the theta energy (p = 0.011). Delta oscillation, which represents the deep rhythmic connection between areas, continually decreased from T1 to T2, and kept decreasing in both groups after T3. The P+K group displayed a significant energy decrease compared with the propofol group (p = 0.019).
Part 2: Inhaled S+K group had a higher gamma T3 bar than the S group (p = 0.18). When sevoflurane is administered at sub-MAC concentrations to achieve surgical levels of general anaesthesia, the EEG shows strong alpha oscillations similar to propofol. In the alpha energy analysis, (Figure 3) both groups had energy increased at T2, but no difference was evident after ketamine was administered (p = 0.8). Sevoflurane, unlike propofol, showed a strong theta energy, which may reflect that it exerts its effects through a non-GABAergic mechanism while patients lose consciousness. The theta energy increased which T2 bars were higher than the T1 bars. Ketamine exhibited a decrease effect on theta energy (p = 0.99). The S+K group showed a decrease effect on delta energy.
Part 3: BIS data comparison between groups are showed in (Table2). The p-value of ANOVA F-test in propofol group is 0.054 and the p-value in sevoflurane group is 0.265; which showed the BIS data has no differences with analgesic dose ketamine weather combined with propofol or sevoflurane.
Table 2: BIS data comparison between groups.
Note: The value of ANOVA F-test is 4.115, with the p=0.054 P+K: propofol + ketamine.
P: propofol.
Note: The value of ANOVA F-test is 1.294, with the p=0.265 S+K: Sevoflurane+ketamine.
S: Sevoflurane.
Discussion
The results of demographic data comparison revealed no significant differences between the four groups. The ANOVA values of all mean frequencies of each IMF’s had negative findings, demonstrating that there were no differences between the four groups of patients based on the enhanced EMD technique. The mean frequencies of IMF1 to IMF6 are equivalent expression compared with EEG in clinical identification. These results support the notion that enhanced EMD can decompose frontal-area EEGs into different IMF’s which represent corresponding oscillations. According to part 1 results, ketamine injected with propofol has a significant gamma stimulation effect (p = 0.02). While gamma oscillations represent that glutamate receptors are active, [16] we know that propofol mainly inhibits GABA-A receptors. We can infer that ketamine binds to NMDA receptors, it blocks the channel and make the downstream glutamate receptor more active, induces signalling and makes the BIS values unpredictable (Flowchart 1). Generally, the channels on inhibitory interneurons are more active than those on pyramidal neurons, [13] so the energy is not influenced by coinjected with propofol. The beta waves showed an energy increase when the patients lost consciousness (T2), and the patients were in the paradoxical activated stage when beta oscillation increased.
Ketamine selectively downregulates GABAergic inhibitory neurons and allows brain regions to continue to communicate but with less modulation and control by the inhibitory interneurons [1]. Therefore, ketamine also sustains beta oscillation. Frontal alpha oscillation of propofol persisted when the patients lost consciousness, indicating that anaesthesia occurs through the thalamocortical loop mechanism. The alpha oscillation persisted at a high level and was not affected by ketamine, which showed that energy gathered in middle- frequency oscillations after the patients lost consciousness (Flowchart 2). Therefore, ketamine did not affect alpha oscillation (p = 0.47). Theta oscillation represents the connection between the hippocampus and frontal lobe, and ketamine had a dissociative effect which inhibited the theta oscillation of propofol (p = 0.018). Delta oscillation represents the deep rhythmic connection between areas of the brain. Propofolinduced delta oscillation likely results from decreased excitatory inputs to the cortex caused by propofol’s inhibition of GABA A-mediated arousal centres in the midbrain, pons, and hypothalamus [1]. The connection may be broken due to ketamine’s dissociative effect (p = 0.019). Decreasing excitatory inputs from the thalamus and brainstem to the cortex enhances hyperpolarization of cortical pyramidal neurons, an effect which favours he dominance of slow wave EEG with predominant delta oscillations.
According to part 2 results, though there are differences between IMF’s (p<0.01 both in S+K and S groups), there are no statistical difference in root mean square energy differences between S+K and S. In both S+K as well as P+K groups show increase in beta and gamma oscillations after ketamine was injected (data was not shown); combine with no change in BIS value in both groups, showed that ketamine at an analgesic dose can mask the alpha oscillations of propofol and sevoflurane. Beta oscillation of propofol might be caused by the temporary blockade of potassium channels, on the basis of the theory that an increase in beta oscillation is due to the effect of sevoflurane on two-pore potassium channels causing hyperpolarization. Interestingly, the energy increase of P+K is higher than that of S+K (p = 0.02, data was not shown); the paradoxical excitation affects beta oscillation more than ketamine at an analgesic dose. Alpha oscillation exhibited a similar response in both the S+K and P+K groups. Different from propofol, sevoflurane showed theta oscillation, which reflects one of its non-GABAergic mechanisms: an HCN slow oscillation effect. Inhibition of HCN1 by anaesthetics in cortical neurons has been demonstrated to contribute to the synaptically mediated slow wave cortical synchronization that accompanies anaesthesia-induced hypnosis [17,18].
Low-dose ketamine did not affect the BIS value in both sevoflurane as well as Propofol group BIS value. Though there is a change in energy or IMF data BIS value is not altered, which confirmed that our method can express more information than tranditional. The results support the notion that EEG energy can be decomposed and calculated. This study had some disadvantages. Frequency fluctuations occurred due to noise, which was amplified after enhanced EMD. The sliding window of the root mean square energy showed there were many noises in the operation room, which increased the confounding factor. We used a BIS monitor as a raw EEG collector, which may have resulted in the loss of some signals because of the bandpass filter. Another limitation is the case number of sevoflurane group, which need increase study numbers to improve confidence in the results.
Conclusion
We prove that the EEG effects on analgesic dose ketamine can be decomposed with enhanced EMD. Further when analgesic doses of ketamine is administered while the patients are anaesthetized with either sevoflurane or propofol while the EEG composition is altered BIS value remains the same. In conclusion, ketamine significantly blocks propofol theta oscillation, which may represent the blocking of the connection between the hippocampus and the frontal lobe. For the same reason, ketamine also blocks the thalamocortical delta oscillation of propofol, implying that it blocks the deep connections between various areas of the brain.
For more Articles on: https://biomedres01.blogspot.com/
No comments:
Post a Comment
Note: Only a member of this blog may post a comment.